Current projects:
Vehicle headlamps play a central role in road safety at night. A precise adjustment of the headlamps and alignment of the light distribution is essential to illuminate the roadway over a sufficient length and width without dazzling oncoming traffic. Only under these conditions can optimal visibility for all road users be ensured. Especially in modern lighting systems with high luminous intensity and adaptive functions, an exact alignment is indispensable in order to fully exploit the safety-relevant advantages while simultaneously complying with legal glare limits.
Headlamp alignment is carried out at the factory during vehicle production, but it changes and shifts during use. Both irreversible influences, such as mechanical wear or repeated jolting during driving – which currently must be compensated by manual readjustment – and temporary influences such as load or suspension changes – which can only be corrected by an automated system during driving – affect the alignment. Even slight deviations in the vertical or horizontal orientation of the light distribution can cause significant changes in visibility and glare conditions.
At the Department of Adaptive Lighting Systems and Visual Processing, an automated procedure for recalibrating headlamps during driving – the so-called Re-Aiming – is therefore being developed. The goal is to continuously monitor the alignment of the light distribution in real-world driving conditions and to automatically correct it when necessary. The targeted accuracy in the range of 0.1 degrees places high demands on the overall system.
For reliable recalibration, suitable methods must be identified and combined. The system must be capable of recognizing and evaluating environmental conditions in which markings projected onto the roadway are visible and can be evaluated. To create these markings, requirements must be defined regarding the resolution and stability of the light distribution, the design of the markings, and the robustness under varying environmental conditions. The markings are detected and analyzed by camera-based sensor systems so that the current alignment can be precisely determined. Based on this information, the light distribution is automatically adjusted using a suitable decision logic.
For system development and validation, both simulation-based environments and prototype implementations are used. Typical driving situations are identified in which Re-Aiming can be reliably performed – for example, under certain road surfaces, lighting conditions, or speeds. In parallel, it is investigated how the findings can be integrated into existing regulations or procedures of periodic technical vehicle inspection.
In the long term, Re-Aiming has the potential not only to improve lighting quality during operation but also to reduce maintenance requirements and the use of mechanical components, which can lead to cost savings. In addition, the process provides impulses for the further development of legal regulations and test methods. The research follows a practice-oriented approach, focusing on system compatibility, feasibility, and a tangible benefit for visibility and safety in road traffic.
You can find the whitepaper here: Download (opens in new tab)
Funded by the German Research Foundation (DFG) – 516682649
This research project focuses on improving the simulation of light in the close vicinity of cultivated plants, which necessitates the optical modeling of these plants. The simulation involves modeling in two key areas: (1) creating a structural model of the plants and (2) establishing an optical model of the plant components. Notably, there have been gaps in the literature, particularly concerning the accuracy of such simulations, with optical properties of deciduous trees and field crops being studied, but not those of plants typically grown in protected environments.
This research project aims to develop an optical structural model of young cucumber plants, which will subsequently be used for virtual testing of optical sensors as an illustrative application. This extends the field of virtual plants from systematic use for a better understanding of plant physiological relationships to employing virtual plants for testing sensors virtually and analyzing the connections between metrics and plant properties in a virtual context. One exemplary application of this approach is phenotyping, where sensor responses to specific plant features can be simulated in advance without the need for the physical existence of such plants.
To achieve this, a precise spectral characterization of the optical elements is essential, and data collection in this regard plays a significant role in the research project. Beyond the primary focus on engineering sciences, this research project also benefits disciplines that generally deal with virtual plants. The deliberate modeling of a model plant, the publication of the model, and the associated data significantly enhance the data available in this field.
Funded by the German Research Foundation (DFG) – 513756113,
the research project 'Multidimensional Color Preference Modeling in the Context of Subjective Lighting Quality' aims to provide a comprehensive description of lighting users' preferences in illuminated spaces. The goal is to enable sustainable and human-centered lighting design from an ergonomic perspective in the future. Existing color and light quality models have been unable to comprehensively and appropriately describe the interaction between preferred color shifts and the lighting parameters of illuminance and color temperature as discussed in the literature.
Therefore, the objective is to conduct a systematic investigation of these relationships based on the established formalism of the ANSI/IES TM-30-20 standard. This investigation will serve as the foundation for deriving a corresponding lighting quality model. The final model will, through a hue-specific approach, be capable of predicting the lighting users' preferences in real spaces based on illuminance and color temperature. The validation of the model in real-world application scenarios is aspired, and all test and validation results will be made available through a dedicated open-access Git repository.
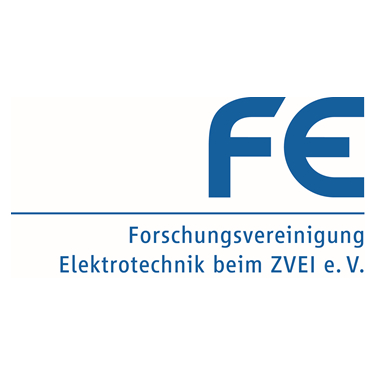
Funded by the Federal Ministry for Economic Affairs and Climate Action (BMWK)
The light-emitting diode (LED) and its continuous development are of high technological and economic significance for the lighting market as a whole, especially for German manufacturers and users. From a technological perspective, LEDs offer high energy efficiency and great flexibility in control compared to conventional light sources. From an economic standpoint, the long lifespan, which can now exceed tens of thousands of hours, is a crucial feature for manufacturers and users. Since 2014, indicating the lifespan on lamps and luminaires has been mandatory, but the actual lifespan often falls short or exceeds the indicated value. This discrepancy poses a significant uncertainty factor, especially for manufacturers in the focus area of this project, which pertains to specialized lighting applications (e.g., street lighting, industrial lighting). It impacts issues like long-term warranty expectations from customers. In practice, this uncertainty often leads to the over-dimensioning of lighting systems and their components, significantly affecting resource efficiency and cost-effectiveness.
The lifespan of a luminaire is the result of a complex interplay of various influencing factors, which has been inadequately researched until now. The design of luminaires and their lifespan calculations are typically based on information provided by component manufacturers. These manufacturers subject components such as LEDs and control devices to accelerated aging tests to extrapolate their lifespan. These tests consider aging at various operating currents and temperatures of the LED chips. In real-world operation, luminaires for the aforementioned applications are subject to particularly demanding conditions, not only due to grid-related requirements (grid overvoltages, grid fluctuations in current, voltage, frequency) but also because of seasonally changing and sometimes adverse environmental conditions, including temperature, UV radiation, vibrations, and humidity.
The foundation for investigating lifespan at the component and luminaire levels under laboratory conditions was laid in the previous IGF projects 'PQL-LED' and 'PQL2'. These projects revealed that, among other things, the influence of ambient humidity had not been adequately studied in the past. Building upon this, the 'PQL3' project expands the common methods for examining component lifespans in the laboratory to include this factor. Furthermore, the insights gained from small-scale laboratory tests must be extended through field trials and statistically verified results. In parallel with the laboratory investigations, a large-scale, innovative field test with over 60 streetlights, in cooperation with the operators of street lighting in the cities of Darmstadt and Frankfurt, will be conducted to obtain real aging data. The collection of data directly in the field on this scale is a novelty. Laboratory experiments will be conducted on sufficiently large samples to subsequently establish aging models. In addition to classical physics-driven modeling methods, data-driven methods from the field of Data Science will be used. A comparison of the classical and data-driven approaches will evaluate whether the use of data-driven methods in future investigations can lead to improved representation of results or even a reduction in experimental effort.
ECSEL Joint Undertaking
With the assistance of Artificial Intelligence (AI), self-learning virtual models known as 'digital twins' of lighting systems are being created. This work is being carried out by 24 industrial and academic partners from eight countries in the European research project AI-TWILIGHT. Two institutes from the Department of Electrical Engineering and Information Technology at TU Darmstadt are also involved in the project. The project has a total budget of 18 million euros and is subsidized with 5.2 million euros from the EU.
The lighting industry has undergone a fundamental transformation with the widespread adoption of LEDs as the standard lighting source. In order for European companies to remain competitive in this altered market in the long term, they need access to modern technologies and research insights from universities. AI-TWILIGHT contributes to this knowledge transfer task. Within the project, physical models are combined with artificial intelligence methods to enhance the lifespan estimation, product development, and maintenance of LED systems. The results of the previous project, Delphi4LED, are also incorporated into this effort. The long-term goal is to create digital twins of lighting systems that will be tested in selected application areas, such as automotive, horticulture, building, and street lighting.
A digital twin is a virtual model of a process or product. Using data, sensors, and algorithms, the 'real' and digital twins communicate with each other. Valuable insights can be gained from comparing the data from both twins. Digital twins are increasingly being utilized, especially in modern production systems of Industry 4.0, which are fully interconnected and self-regulating. The digital twins planned for the AI-TWILIGHT research project will record data during their lifecycle to enable predictive maintenance and product adjustments for subsequent generations of lighting systems.
The researchers at the Department of Adaptive Lighting Systems and Visual Processing (FG Lichttechnik) have extensive expertise in LED systems and components. In the context of the research project, they will initially develop and test procedures for accelerating standardized lifetime tests since current LED lifetime tests are very time and cost-intensive. Furthermore, existing test methods will be expanded to include additional influencing factors such as current, temperature, or humidity for different application areas to enhance the accuracy and reliability of lifetime predictions.
Simultaneously, at the Department of Computational Electromagnetic Field Simulation (CEM), physical models will be combined with machine learning methods to improve the accuracy and reliability of lifetime predictions.
“Through the collaboration of both research groups, knowledge about the physical laws and dependencies of LED systems can be linked with artificial intelligence methods,” says Professor Tran Quoc Khanh from FG Lichttechnik. Professor Sebastian Schöps from CEM explains the added value of this combination: "This allows for the development of optimal lifetime tests and predictions using statistical methods. In practice, this reduces the time and cost involved in testing while maintaining the accuracy of the results.
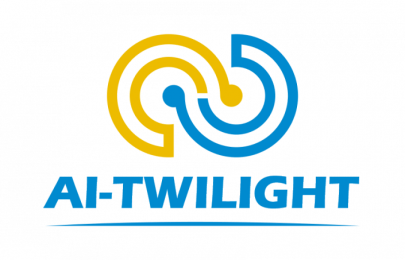
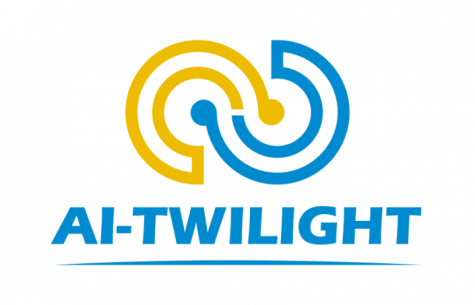
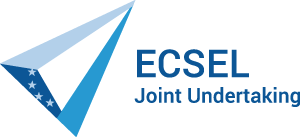
This Project is supported by the Federal Ministry for Economic Affairs and Climate Action (BMWK) on the basis of a decision by the German Bundestag
The ZIM project “Plant-Centric-Lighting” (PCL) aims to develop an adaptable system for energy-efficient and growth-promoting plant lighting. Given the growing population and shrinking agricultural areas, it is crucial to make agriculture more efficient. Greenhouses, and especially innovative concepts like “Vertical Farming” and “Plant Factories with Artificial Lighting” (PFAL), offer solutions to this problem, but there is optimization potential in the lighting systems. The luminaires currently used in greenhouses are often produced with a fixed spectrum and do not consider the varying light optimization potential of plants during their growth.
Within this project, various innovative approaches are combined to increase the efficiency of artificial lighting and optimize plant growth. This includes adapting the irradiation area to the plant geometry, optimizing the wavelength spectrum for growth processes and substance formation, using sensors to capture morphological parameters to determine plant architecture, as well as substances and intelligent control systems. The companies Pracht and Chips4Light are working together with the departments of Biology and the Department for Adaptive Lighting Systems and Visual Processing (FG ALSVV) at TU Darmstadt to implement this concept.
At FG ALSVV, the use of sensors for monitoring and supplementation of natural light, sensors for monitoring plant geometry and determining the content of desired substances, as well as a data-driven control system for continuous adjustment of lighting parameters to biological processes and growth situations, is being researched. This involves the use of multispectral sensors and cameras, as well as machine learning methodologies for processing the captured data and deep learning algorithms for computer vision-based processing of the camera recordings.
The research of the various approaches concludes with the goal of developing a prototypical lighting setup.
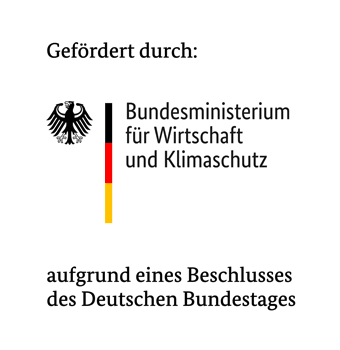
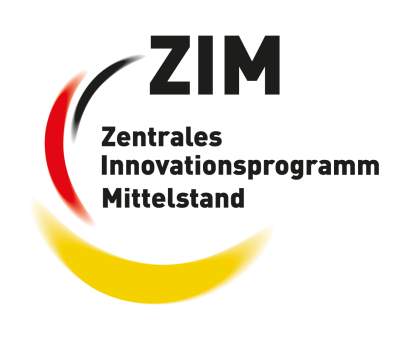
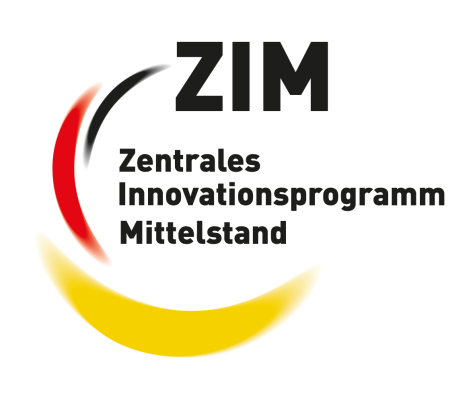
Funded by the Central Innovation Program for SMEs (ZIM).
Within the ZIM project 'smartPBR,' supported by the Federal Ministry for Economic Affairs and Climate Action, an intelligent and highly efficient algae photobioreactor (PBR) is being developed. Project partners, including Algoliner GmbH & Co. KG and the Department of Adaptive Lighting Systems and Visual Processing at TU Darmstadt, aim to contribute to a circular economy where the industrial byproduct CO2 is transformed into a valuable raw material.
The goal of the smartPBR project is to enhance the performance of current algae reactors by developing sensor systems capable of measuring algae density, LED illumination, and current sunlight, subsequently maximizing energy efficiency. Collaboratively, we can minimize energy consumption per kilogram of biomass and reduce costs by integrating this sensor system with predictions of sunlight exposure and energy costs, using an algae growth model.
Microalgae have a wide range of applications, including the production of superfoods, skincare products, pharmaceuticals, and fertilizers. They can also serve as raw materials for bioplastics and provide an alternative source of energy to non-renewable fossil fuels. Microalgae, due to their ability to sequester CO2, offer an attractive and long-term alternative for reducing industrial greenhouse gas emissions. In light of climate change and ongoing global population growth, the development of algae in PBRs has the potential to efficiently and sustainably meet the demand for food, animal feed, polymers, and energy generation in the future.
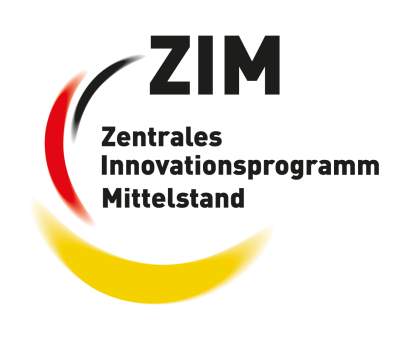
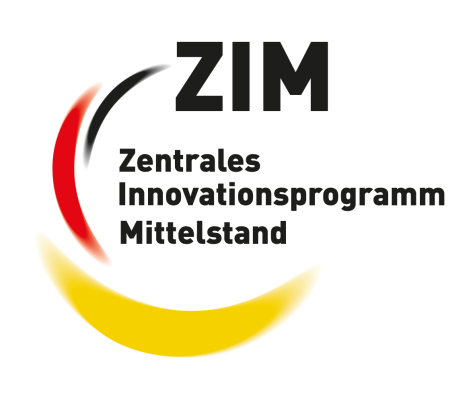
Funded by the German Research Foundation (DFG) – 445336968
The current research project deals with developing an AI-based approach to the determination of dynamically changing, user-oriented luminaire control curves based on continuously recorded sensor input values to realize HCL-compliant system solutions for LED interior lighting. The term “control curve” can be used to summarize all those parameters that can be varied for each luminaire largely independently of one another and with time. For the planned investigations, these are the horizontal illuminance Eh generated on the work surface, the proportion of indirect light in this illuminance (according to Houser), the most similar color temperature (CCT), and the indirect blue light proportion, which significantly defines the physiologically activating component of the lighting and is to be described in the present research project with the help of the circadian stimulus (CS) value (according to Rea and Figueiro).
To determine optimal, AI-based control curves, test subject tests are carried out at different times of the day to determine the users' respective individual light preferences depending on both subjective-psychological and external influencing factors and to correlate them with the corresponding sensor data with the help of machine learning methods. The aim is to develop a lighting system that, based on the incoming sensor data and using an underlying user preference model, can predict the optimal control curve for the current situation and dynamically adjust the room's lighting accordingly over the course of the day.
As a starting point for the implementation of the planned research project, support points of individual control curves are initially recorded by recording user preferences related to the current light situation in the room at different times over several working weeks as part of a first test person study and using AI-supported evaluation with the simultaneously recorded sensor data Relation to be set. Based on this data, the support points determined in this way are summarized in individual, characteristic clusters depending on the ambient conditions as well as the subject-specific influencing factors (e.g., age, gender, chronotype, current state of mind, etc.), which correspond to similar lighting preferences of the test persons. In a second iteration step, these clustered support points are then presented to the participating test subjects in a further test subject study and further adapted to the individual preference using various setting options made available to the test subjects. Together with the continuously recorded sensor data, these additional input values further optimize the control curves. In the third part, a physiological evaluation of these optimal control curves in terms of maximizing user preference is to be carried out in the context of a further study by test persons to answer the question of their activating effect and finally to derive a corresponding user preference model as the basis for future, intelligent lighting systems.
Funded by the Deutsche Forschungsgemeinschaft (DFG, German Research Foundation) – 450942921
The research project deals with the development of an AI-based control of the automotive light distribution to generate situationally optimized, dynamic light distributions. The aim of this research project is to break away from the conventional division of light distributions into low beam and high beam and to present holistic light distributions. In order to develop these light distributions, extensive studies will be carried out to record the current traffic area. Here, representative data for the German traffic area will be recorded first. The aim of the planned drives is to record the German traffic area in its entirety, taking into account different road classes analogous to their real occurrence. For the evaluation, the latest algorithms for the recognition of objects are trained individually and geometric distributions of different objects, cars, trucks, buses (each driving and parking), traffic signs (depending on class), pedestrians, cyclists are created. From these object distribution data as well as other recorded data such as speed, road class, road conditions etc. different traffic situations are automatically created. In addition to the recording of the German traffic area, contrast investigations and luminance analyses in the different road classes are carried out. These serve to determine the safety-relevant light requirements for motor vehicle drivers and to record the current light conditions in the German traffic area. Not only the recognizability distance and the necessary contrast are considered, but also the perception of brightness and homogeneity of the foreground illumination. This is essential, since in addition to an objective increase in safety, the subjective perception of safety also has a strong influence on the driver's well-being and behavior. The results of these investigations are also incorporated into the optimization of the situation-dependent light distributions. In addition, a further study records the gaze behavior of the drivers and calculates optimized light distributions by combining the object distributions and the gaze behavior in road traffic. These theoretical light distributions are then validated. In a first step, the various light distributions are displayed in a driving simulator, independent of the technology used, in the various virtually generated traffic situations. The acceptance and the feeling of safety of the test persons is tested virtually when using the different light distributions. In addition, a further study will test the extent to which drivers' visibility changes as a result of the newly generated light distributions.
Funded by the German Research Foundation (DFG) – 450636577
The eye's pupil diameter is an essential factor in photometric and visual examinations due to its direct influence on retinal illuminance and retinal image quality. A smaller pupil diameter can ensure a more considerable depth of field and a reduction in optical aberrations of the lens, which can positively affect the eye's visual acuity. Various studies show that the optimal pupil diameter for visual tasks in the photopic luminance range is between two and three millimeters. With today's technology of multi-channel LED lights, it is possible to optimize light spectra to directly influence the pupil opening, color and brightness perception, or other visual metrics. The number of different LED color channels in such a system determines the wheel of freedom, allowing visual metrics to be kept constant while others can be changed. The first step towards actively optimizing the pupil opening through interior lighting without influencing other imaging visual parameters is developing a valid pupil model that predicts the spectral and time-dependent pupil diameter of humans. To characterize and model the human pupil behavior, a temperature-controlled 15-channel LED lamp was developed at the lighting technology department (see Figure 1).
Existing pupil models are based on the L- and M-cones' sensitivity in the retina, making them unsuitable for achieving an ideal pupil opening in a light spectrum optimization process [1]. Using these models would make it impossible to vary the pupil diameter while maintaining the brightness and color perception parameters. There is currently a significant prediction inaccuracy when using these pupil models due to the time independence and luminance use as a V (λ) -weighted photometric parameter. The integration of a V (λ) -weighted photometric variable in a pupil model leads to significant prediction errors of the pupil diameter when using multi-channel LED lights as a light source [1] (see Figure 2). This prediction error's main reason is the wavelength-dependent sensitivity curve of the pupil, which does not correspond to the V (λ) curve alone [1,2]. This effect was only understood by discovering the intrinsically photosensitive ganglion cells (ipRGCs), which contributed to the fundamental understanding of stimulus processing in the retina, the perception of brightness, the circadian rhythm, and the pupillary light reaction. These neurophysiological findings have so far been insufficiently integrated into the existing pupil models. The consequence of this are prediction errors of the absolute pupil diameter of current pupil models (see Figure 2).
In our funded DFG research project, we aim to develop a new pupil model based on deep learning, which considers the time dependency and the adaptive weighting of the retinal receptors to control pupil behavior. The latest findings on the mechanism of pupil behavior are to be covered with this model. Specifically, we would like to combine existing time-variant and time-invariant model approaches with a data-driven, non-parametric model to develop an overall model of pupillary behavior. With this, we take into account the dynamic and spectrally dependent receptor adaptation of the phasic and tonic pupillary behavior. The non-parametric model approach ensures a constant improvement in the model quality as the database increases. This is to be achieved through a publicly accessible pupil database that can also be edited by external working groups. The basic model and the basic data should be developed in this research project employing extensive empirical pupil examinations and serve as a general impetus for this generic model project.
The concept for developing a pupil model, which integrates the current findings from basic neurophysiological research, would enable the time-dependent calculation of the pupil diameter for different light spectra for the first time. The accurate calculation of the spectrally and time-dependent pupil diameter is essential in visual experiments, eye safety calculations, and medical diagnostics. Such a model can also help to show the relationship between the perception of brightness and the pupil control path [3].
References
[1] | Zandi B, Klabes J, Khanh TQ. Prediction accuracy of L- and M-cone based human pupil light models. Sci Rep 2020;10:10988. https://doi.org/10.1038/s41598-020-67593-3. |
[2] | Zandi B, Kunst K, Khanh TQ. Einfluss der melanopsinhaltigen Ganglienzellen auf die kurz- und langzeitige Pupillenlichtreaktion. 120. Jahrestagung der Dtsch. Gesellschaft für Angew. Opt., Darmstadt: 2019. |
[3] | Zandi B, Guo X, Bodrogi P, Khanh TQ. EXPERIMENTAL EVALUATION OF DIFFERENT BRIGHTNESS PERCEPTION MODELS BASED ON HUMAN PUPIL LIGHT RESPONSES. Proc. CIE 2018 Top. Conf. SMART Light., vol. 2, International Commission on Illumination, CIE; 2018, p. 201–8. https://doi.org/10.25039/x45.2018.OP34. |
Funded by the Deutsche Forschungsgemeinschaft (DFG, German Research Foundation) – 500805487
Project actuator technology
At the Laboratory of Adaptive Lighting Systems and Visual Processing, multi-channel LED luminaires are built using chip-on-board LED technology. A chip-on-board LED contains 9 wavelengths (360 nm, 405 nm, 450 nm, 520 nm, 600 nm, 660 nm, 730 nm, warm white and 900 nm). For this purpose, a special driver is designed for the chip-on-board LEDs. The features of this driver are wide frequency range (100 Hz – 20 Khz), current (100 – 750 mA) and duty cycle (10 – 98%). All LED chips are controlled separately. A human machine interface (HMI) software is also programmed. In addition, the wavelength light lines for the different wavelengths are designed, simulated and implemented. The finished lights will be characterized with a measurement system (U-ball, Peltier module, Thorlab devices, spectrometer). Consequently, the intensities (1200, 600, 300, 200, 100, 50 µmol/ m2s = 6 photon densities), wavelength, clocking and the combinations of the multi-channel LED lights for photosynthesis research are managed. In the Applied Plant Science Laboratory, the suitable plant species are cultivated and researched with the generated light conditions. The research variables are a total of 6 spots x 6 frequencies x 9 radiation spectra x 6 intensities.
Project sensor technology
Leaf temperature is measured with two temperature sensors and infra-red camera. The leaf color is determined by spectral reflectance. A very important work chain is the “Multivariate Fluorescence – based Prediction – Model”. The aim of this work chain is to represent the photosynthetic rate determined by gas exchange analysis as a function of the parameters determined by chlorophyll fluorescence analysis, leaf reflectance and leaf absorption data, and leaf temperature determined by thermography. It is similar to the study by Losciale et al. (https://doi.org/10.1104/pp.113.225243), but with a much larger number of input parameters and more focus on the effects of the light factor. The above parameters are measured simultaneously: Photosynthetic rate by gas exchange analysis with a gas exchange system, fluorescence analysis with an imaging PAM system, temperature, reflectance, and absorbance data as described in the paper so cited above. Both the imaging PAM system and the gas exchange system are available in the APS working group.
Modeling = photosynthesis as light – function
Photosynthesis will be analyzed separately for each plant species. Then, the data at each growth stage will be considered, since it can be assumed that the leaf property (reflection, absorption, leaf thickness, leaf texture) changes with the growth stage. At each point along the time axis of the growth process, exemplary wavelengths (e.g., 450 nm and 660 nm) and two current levels will be used to analyze the photosynthetic rate as a function of frequency and duty cycle. Two possible solutions emerge. If the photosynthesis rate is independent of frequency and duty cycles, the results of all frequencies and duty cycles are combined. If the photosynthetic rates are dependent on frequency or duty cycle after a statistical significance test, the frequencies and duty cycles are considered as independent variables. The data are then grouped into different frequencies and duty cycles. An energy consideration for some desired photosynthetic rates will be performed at this point. From this, a range of preferred frequencies and duty cycles can be determined from an energy and economic perspective. The number of parameters can thus be reduced. What remains are the parameters of leaf temperature, wavelengths, and irradiances for each plant species at each defined growth time.
At each leaf temperature, the photosynthetic rate can be plotted as a function of wavelength and radiation intensity. At different leaf temperatures, there are different functions and at different growth stages, there is a set of functions. Thus, the required photosynthetic rate can be found by entering the vector V = f (leaf temperature, wavelength, radiation intensity, frequency) into the data structure.
Data collection and storage form the basis for tapping previously unused innovation potential for lighting systems with new use cases. However, the luminaires or lighting systems, including the associated sensors, must record data, transfer them to a cloud with uniform semantics and save them. In this project, the data from test systems installed across 14 locations are brought together in a common IoL cloud. This project aims to analyze the sensor and luminaire data generated in the test systems while simultaneously testing the architecture for data storage and data transmission. Building on this, the centrally stored data is analyzed and evaluated, and data-driven lighting use cases are examined and processed.
The leading research questions for the “Internet of Light” project as a joint project between the German lighting industry and the research institutions are:
- How can the data generated by sensors and lights in the lighting systems be transmitted, persisted, and analyzed in a uniform, clearly structured data format efficiently and with low latency in one or more cloud systems?
- How do you deal with transmission failures, physically implausible data, and restrictions concerning the system?
- Which sensor types and operating data are required for data-driven use cases in different lighting systems?
- What mutual interactions, influences, and correlations do the luminaire and sensor data have with one another?
- What benefits can users and system operators gain from the luminaire and sensor data over longer periods of several seasons, for example, to optimize and prove economic efficiency, environmental friendliness, and user-friendliness under real laboratory conditions and for later field systems?
- Which algorithms and methods can be used for processing sensor data in the field of lighting technology?
Visual Efficiency – A sustainable evaluation scale for modern architectural and lighting design
Current architectural lighting design covers only the minimal values given by legal norms securing the possibility of visual tasks. This leads to a lack of acceptance, reduced well-being and sustainability. Our approach introduces a new evaluation scale called “Visual Efficiency” that expands the classical pure visual evaluation by including psychophysical and psychological factors, user preferences as well as energy efficiency. To develop such a new evaluation scale, field studies intended to provide access to both quantitative and semantic rating data of architectural lighting situations will be conducted.
Eventually, this will pave the way for achieving the vision of a general architectural lighting guide that includes all requirements to generate well-being for the user and offers a versatile, sustainable and efficient usage.
The project is part of a collaboration with the FG Entwerfen und Gebäudetechnologie of FB 15 (Architecture).